Interfacial Microbial Engineering
Abstract
The cell surface of all cells, including microorganisms, contains a variety of functional biomacromolecules, such as proteins and polysaccharides, which are responsible for the exchange of substances and information with the external environment, as well as interactions with interfaces and other cells. In other words, the cell surface layer serves as a platform for functional biopolymers. The cytoplasm, which contains major cellular components, is enclosed by a lipid bilayer membrane. However, most microbial cells also have a cell wall on the exterior that maintains the cell's mechanical strength. Bacteria are classified into Gram-positive and Gram-negative bacteria based on differences in cell surface structure. The cell membrane of the former is covered with a thick cell wall composed mainly of polysaccharides called peptidoglycans, while the latter has another lipid bilayer called the outer membrane outside the cell membrane. The space between the cell membrane (inner membrane) and the outer membrane is known as the periplasm, where a thin peptidoglycan layer exists. E. coli, the most important bacterium in research and industry, is a Gram-negative bacterium, while B. subtilis is a Gram-positive bacterium.
Microorganisms house a wide variety of enzymes within their cells and also secrete them outside their cells. These enzymes are used in the production of fermented foods, pharmaceuticals, chemical products, biofuels, and in environmental applications such as wastewater treatment, deodorization, and bioremediation. In wastewater treatment, microbial cells absorb organic matter, a component of dirt, and mineralize it through catabolic metabolism. In any case, the membrane transport of enzymes and substrates is an essential step in a series of microbial reactions. Additionally, microorganisms in the environment adhere to solid surfaces and form complex microbial communities called biofilms (see below). Within these communities, microbial cells grow by exchanging substances and information. Here, the surface layer of microbial cells plays a vital role in adhesion, cell aggregation, and substance and information exchange.
In our laboratory, we conduct fundamental research on the structure, function, and interaction of microorganisms with the keywords "Microorganism," "Cell surface," and "Interface." Based on this research, we aim to establish "interfacial Microbial Engineering" to construct new methodologies and technologies that will lead to green innovation. By elucidating the surface structure and function of microbial cells at the molecular level and modifying and designing the cell surface accordingly, we work to develop novel technologies for the immobilization of microorganisms, presentation of the cell surface, and control of material conversion rates. We also analyze the interaction of microbial cells and cell surface structures with interfaces to develop technologies for controlling microbial adhesion and biofilm formation. Simultaneously, we are developing technologies for utilizing biofilms in wastewater treatment.
Summary of achievements
Acinetobacter sp. Tol 5, a toluene-degrading bacterium obtained from a flue gas treatment plant, has a fantastic adhesive property, so much so that cells coat the inner walls of pipettes and plastic tubes just by sampling. It also exhibits cell self-aggregation: when a polyurethane sponge is placed in a cell suspension of Tol 5, almost all cells adhere to the surface of the polyurethane fiber-forming cell aggregates within a minute of contact (Fig. 1). This high adhesiveness is due to the fibrous structure covering the cell surface layer (Fig. 2). These are collectively called bacterio-nanofibers. To date, Tol 5 has been shown to have at least three types of perichaetial nanofibers made of proteins. Microbial cells are excellent biocatalysts. They can be used to produce a variety of valuable chemicals and biofuels and have been considered a trump card for green technology. However, their practical application has been limited due to many difficulties in cost and realization of stable processes. Professor Hori has succeeded in developing an innovative microbial immobilization technology using bacterial nanofibers. This will enable the continuous and repeated use of expensive microbial catalysts and dramatically improve the operability, convenience, and stability of bioprocesses.
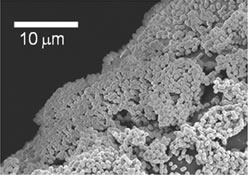
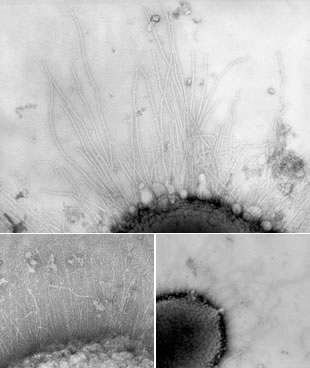